Superimaging
About the PI’s:
Dr. Vitalii Silin, the PI, has over 25 years of experience in physics, optics, SPR, preparation of thin films, and development of mathematics/software specific to SPR microscopy. He has designed and built detectors for making precise measurements of neutron reflectivity, SPR, and EIS. More recently, he has built two generations of SPRM instruments, which form the basis for the Q-SPRM. The PI will oversee the entire build project and coordinate the biological experiments with Dr. Nelson's group. Dr. Daniel Nelson, the co-PI, has 15 years of experience in protein biochemistry, molecular biology, microbiology, and bacteriophage biology. His group has expertise in studying phage-host interactions and will provide all biological samples used in proof-of-principle imaging experiments with the Q-SPRM.
About Q-SPRM:
Despite the fact that optical microscopy has significantly progressed in improving resolution and contrast, it has not yet achieved the resolution needed for biomolecular imaging. Moreover, most imaging techniques require labeling that may interfere with processes inside a living organism. Many optical techniques may be utilized to work toward refining microscopy to approach the diffraction limit of electron microscopy in living systems (see Table 1.). One of them is the surface plasmon resonance based optical microscopy (SPRM) that has been developed over the last 20 years. However, to our knowledge, at least two factors limit the breadth of SPRM applications. First, to date, SPRM has only been applied to objects adsorbed to the surface plasmon (SP) supported surface – i.e., at the metal surface of the cover slips. This significantly limits the capabilities of SPRM for imaging objects in their native environment. For example, it is difficult to image the slices of tissue or the surface of the live cell on the side not bound to the surface. To overcome this limitation, we propose to study the interaction of the evanescent field that is produced at the focal point on the cover slip surface with the object not bound to the surface of the cover slip. Second, we believe that the contrast and resolution of SPRM is not yet optimized. Our proposed studies on a new quantitative SPR microscope, or Q-SPRM, will contribute significantly to the understanding of the problems and help to develop solutions. Moreover, SPRM and a recently described imaging method that uses arrays of plasmonic prolate spheroidal noble metal nanoparticles, strongly suggest that sub-wavelength resolution of SPRM imaging with high contrast and without the need of labels could be achieved.
Type | Q-SPRM | PALM/STORM | STED | NSOM | AFM | EM |
Max lateral resolution | expected |
~20 nm | ~20 nm | ~20 nm | ~5 Å | ~0.5Å |
Axial resolution | ~1 Å | Single molecules | ~45 nm | ~2 nm | ~5 Å | ~0.5 Å |
Temporal resolution | msec | sec | sec | NA | NA | NA |
Contrast | Up to 10-9 refractive index | fluorescence | fluorescence | enhancement by: staining, fluorescence labeling, phase, illumination | NA | Staining |
Sample Biological Applications | Virus and bacteriophage interactions with cells; mitochondria; transmembrane proteins in phospholipid layers; synaptic vesicles | Single particles/ molecules tracing, lysosomes, mitochondria Membrane protein distribution in live fibroblast, Virus particles, etc. | Synaptic vesicles, Nuclear protein slicing and localization | Nanoscale organization of membrane proteins, cell’s surface | 3-D images of individual biomolecules and small biomolecular complexes. Mapping mechanical properties of live cells, phospholipid membranes, etc. | Fixed proteins, bacteriophage and viruses etc. |

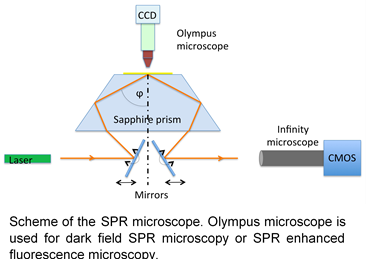
